Indo-French Biomolecular NMR Winter School: 14-20 Dec 2024
Our research focuses on understanding the link between the biomacromolecular conformational dynamics occurring on different lengths and time scales and their concerted role in biological function. In parallel to NMR method developments for biologically exciting problems, we use a combination of proton-detected solid- and solution-state nuclear magnetic resonance (NMR) and molecular dynamics (MD) simulations to elucidate the interplay between the functional active-site conformational changes, ligand binding events and allosteric mechanisms. Our long-term interest is to integrate protein dynamics knowledge with the structure and dynamics-guided evolution for a detailed understanding of the function and tailored interference with pharmacological targets.
Our research interests include the following topics:
-
Investigating the Protein Dynamics, Interactions and Allostery for Therapeutic Potential.
-
Protein Engineering for Nucleic Acids Modifications.
-
Investigating the Functions of Larger Biomolecules (100 kDa and beyond) by NMR: Allostery and Binding Networks.
-
Understanding the Behaviour of Proteins Linked to Brain Disorder.
-
Nuclear Magnetic Resonance (NMR) Method Developments (solution-, solid-state and high-pressure NMR) and Applications.
-
Developing Carbon Capture and Conversion (CCC) Technologies.
We work into three parallel research areas, one focusing on the basic biomedical, the other on the industrial-application-based research projects, and the third one focusing on the direction of NMR method developments for interesting biological applications.
Research areas:
Molecular Biophysics, Biological Chemistry, Structural Biology, Industrial Biotechnology, and Biomolecular NMR spectroscopy
Research focus and interests:
,
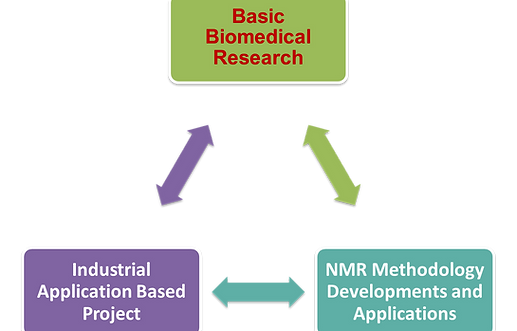
We work into three parallel research areas, one focusing on the basic biomedical, the other on the industrial-application-based research projects, and the third one focusing on the direction of NMR method developments for interesting biological applications.
Molecular Biophysics, Biological Chemistry, Structural Biology, Industrial Biotechnology, and Biomolecular NMR spectroscopy

Research areas:
Proteins are highly dynamic molecular machines sampling various inter-converting conformations that determine their functionality, ligand binding, and allosteric properties. Allosteric interactions, an intrinsic property of proteins, can be triggered by protein-protein/ligand binding, mutations, and post-translational modifications happening remotely from the active site. Protein dynamics govern allosteric communication between specific sites, which is central to regulating function. It is imperative to unravel the link between protein structure and dynamics and design allosteric modulators to control their function. The extensive ranges of sampled timescales and sparsely populated intermediate conformational states have greatly challenged many biophysical methods. In this context, NMR spectroscopy has emerged as a unique and robust method for investigating biomolecular dynamics at atomic resolution, which is strength and expertise of the Singh group. NMR methods can simultaneously probe the motions, exchange rates, lifetimes, populations and their structures, allowing an in-depth understanding of protein interactions and permitting targeted interference by pharmaceuticals. In addition, it yields direct information on physical (protein-protein interaction, ligand-binding) and chemical (mutations, post-translational modification) events to individual amino acids. This method has been applied to investigate critical biological systems, establishing a solid link between dynamics and function in healthy and diseased states. Recently (Singh et al., 2019 JACS, Singh et al., 2020 Angew Chem), we have adopted a concerted use of NMR and MD simulations to elucidate the interplay between the functional active-site conformational changes and associated water dynamics in the pocket of an important drug target.
We plan to investigate the collective and site-specific dynamics of intrinsically disordered and globular proteins and link them to their biological function and allosteric control mechanisms. We want to understand how protein dynamics knowledge might be used to design mutations in a protein to remodel its ensemble of conformational states. At the end of the projects, we will comprehensively understand the protein dynamics design and coupling to allostery. This study may translate into designing effective allosteric drugs targeting aberrant protein conformations involved in various deadly human diseases such as cancer and neurological disorders.
Most current and past research work characterize protein structure, dynamics, and protein-drug interactions. We are assessing in detail for various protein targets the link between the conformational changes occurring on different length and time scales and their function. In the recent articles8-9, we used proton-detected solid- and solution-state NMR and MD simulations to elucidate the interplay between the functional active-site conformational changes and associated water dynamics in the pocket of human carbonic anhydrase II. A changing geometry of the coupled protein-water network, a previously elusive dynamics, was an essential aspect for functionality, explaining the feasibility of substrate/product exchange and proton transport out of the pocket that was erroneously believed to be rigid previously. In parallel to the solution and solid-state nuclear magnetic resonance (NMR) method developments for biologically exciting problems, my long-term interest is to integrate protein dynamics with the structure for a detailed understanding of the function and allow tailored interference with pharmacological targets.
Current and past research contributions are summarized in the following paragraphs.
Current and past research contributions:
We have reported that acetate assimilation in C. reinhardtii does not directly lead to TAG formation but via bicarbonate/CO2aq pathways. Photoautotrophic mode is the best growth condition for starch and lipids (mainly triacylglycerols, TAGs) in C. reinhardtii (Singh et al., 2014, PloS One, 9, e106457). Besides, we also delineated an excellent cellular system for probing the nitrogen starvation, nitrogen uptake and the consequent changes in carbon flow concerning both starch/lipid accumulation and their re-mobilisation and the changes in free amino acid pools in C. reinhardtii cells (Singh et al., 2016, Algal Research, 18, 33-44).

(1) Regulation of starch, lipids and amino acids upon nitrogen sensing in Chlamydomonas reinhardtii:
We have reported that the DNA endonuclease activity of UVI31+ is facilitated by the catalytic triad residues involving Ser114, His95 and Thr116. Further, decreased endonuclease activity of the S114A mutant is consistent with the direct participation of Ser114 in the catalysis. This study revealed the first structural description of a plant chloroplast endonuclease regulated by UV-stress response in C. reinhardtii algal plant (Rout# and Singh# et al., 2018, Scientific reports, 8, 13750, # first co-authors).

(2) Structural characterisation of a novel KH-domain containing plant chloroplast endonuclease:
We have shown the potential of flagella from green algae to align the biomolecules under a magnetic field weakly. The flagella are rod-like structures of ~500 nm in diameter and 5-15 µm long, and nine doublet microtubules highly stabilize the flagella. This highly stable structure makes flagella quite suitable for bio-molecular NMR measurements over a wide range of temperatures, low pH, high ionic strength, or in the presence of detergents. Thus, this method provides a robust and cost-effective alternative to align biomolecules in a magnetic field and measure residual dipolar couplings useful in structure determination, refinement and validation (Singh et al., 2013, Chemical Communications, 97, 11403-11405).

(3) Flagella as a novel alignment medium for the measurement of residual dipolar couplings in proteins:
Solid-state NMR has been employed to characterise a broad range of bio-macromolecules and supramolecular assemblies. However, the individual monomeric units' size has rarely exceeded 15 kDa because of limitations in sensitivity and resolution. As such, enzymes, often more complex and comprise long peptide chains, have not been easily accessible, even though solid-state NMR studies could provide manifold desirable information. We have demonstrated more than 1200 backbone and side-chain chemical shifts can be reliably assessed from minimal sample quantities (~0.5 mg) for a 29 kDa human enzyme of the carbonic anhydrase family, giving access to its backbone dynamics and intermolecular interactions with a small-molecule inhibitor. The possibility of a comprehensive assessment of enzymes in this molecular-weight regime without molecular-tumbling derived limitations enables the study of residue-specific properties essential for their mode of action as well as for pharmacological interference in this and many other enzymes (Vasa# and Singh# et al., 2018, JPC Letters, 9 (# first co-authors), 1307-1311 and Vasa, Singh et al., 2019, Angew Chem, 58, 5758 –5762).
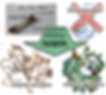
(4) Structural characterisation of Human Carbonic Anhydrase II and proton-detected solid-state NMR method developments:
Hydration water plays a vital role in the structure-dynamics-function relationships of many biomolecules. However, the specific links between protein-bound water molecules and biological function often remain elusive—the challenges of characterising the coupled protein-water dynamics and associated thermodynamics in atomic detail. Dynamics in the active centre of enzymes are essential to their functionality. Many enzymes also have well-defined networks of hydrogen-bonded water molecules, the location of which is defined by H-bond donors and acceptors on the protein surface. However, since these molecules' residence time at the respective site is short (ns), it is not intuitive that protein movement on the microsecond time scale can affect the water network. In recent articles (Singh et al., 2019, JACS, 141, 49, 19276-19288 and Singh et al., Angew Chem., 2020, 59, 22916-22921), proton-detected solid- and solution-state NMR and MD simulations probe the structural water lifetime in the active pocket of human carbonic anhydrase II, which is on the 100 µs time scale. The network's changing geometry could be important for exchanging substrate/product, especially the kinetically "difficult" step of transporting a proton out of the active pocket. We observe conformational dynamics of the active site in solution and crystalline states. The findings will fundamentally impact current interpretations of the hCAII structural, kinetic and thermodynamic data. And will facilitate improved concepts for more potent enzyme inhibitors and ligand-binding thermodynamics.

(5) Microsecond-timescale dynamics of the functional water in the active site of an enzyme:
Some of our lowest energy NMR protein structures

Deposited NMR protein structures: